Navigating In Vivo Bioluminescence Imaging: Tips for Enhanced Success and Efficiency
Simon Moe, Promega Corporation
Publication date: March 2024
Introduction
Optical imaging is a powerful tool that can help researchers observe biological events in real time. The ability to clearly visualize protein behaviors, intricate chemical reactions and the dynamic interplay of internal processes as they happen is key to unraveling these complex biological activities. In vivo optical imaging is particularly useful for tracking gene activity, protein interactions and specific cell groups within small animals, providing insights that advance our understanding of biology. The two primary methods of in vivo optical imaging are fluorescence and bioluminescence.
Fluorescence imaging relies on light-activated molecules that are typically tagged to a protein of interest. Upon excitation using an external light source (a lamp, LED or laser), these fluorophore molecules emit light at a slightly longer wavelength, which can be detected using a camera or sensor (Refaat et al., 2022). Bioluminescent imaging (BLI) relies on visible light emission in living organisms without the need for any external light source. Bioluminescence is a naturally occurring phenomenon that was modified to explore biological processes for in vivo monitoring. BLI uses one of several luciferase enzymes that will oxidize a substrate (ex. Luciferin), which in turn generates light (Love & Prescher, 2020). As shown in Figure 1, cells of interest can be injected into an animal or a mouse with a luciferase transgene, which can be used in conjunction with luciferase substrates. The advantage of BLI rests on the fact that no external light is required to generate bioluminescence, allowing visualization into tissues of an animal with a greater signal:noise ratio and less autofluorescence. This provides a highly sensitive and quantitative method for measuring biological processes in live animals.
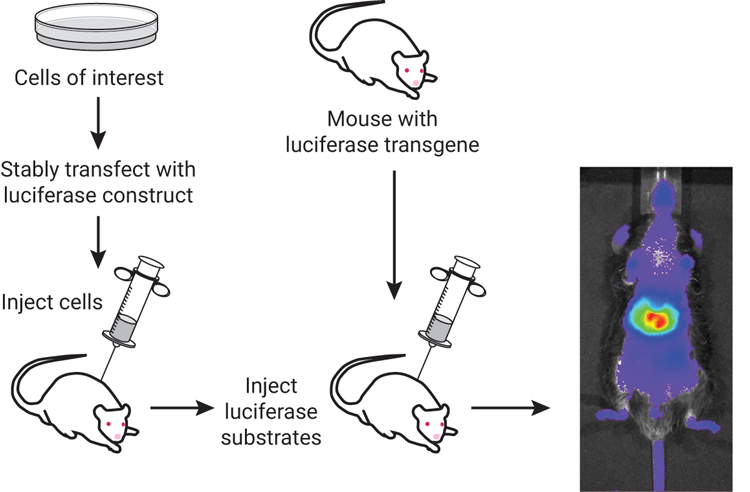
Luminescent Reporters and Substrates
Reporter | Substrate |
---|---|
Firefly luciferase (FLuc) | VivoGlo™ Luciferin, In Vivo Grade |
NanoLuc (NLuc) | Nano-Glo® Fluorofurimazine In Vivo Substrate (FFz) |
Renilla (RLuc) | ViviRen™ In Vivo Renilla Luciferase Substrate |
Firefly (FLuc)
Nanoluc (NLuc)
NanoLuc® luciferase (NLuc) is a small (19kDa) reporter that is ATP-independent and uses furimazine as a substrate. NLuc emits blue light at roughly 460 nm. When compared to other commonly used reporters, NLuc is very bright and sensitive. This extreme brightness means that exposure times can be reduced to only a few seconds, whereas other reporters require minutes of exposure. NLuc’s small size makes it ideal as a viral reporter or protein fusion partner. Due to its ATP independence, NLuc can assist in observing events both inside and outside of the cell. The Nano-Glo® Fluorofurimazine In Vivo Substrate (FFz) provides a method to observe NanoLuc expression inside living small animals. The strength of the FFz substrate is expanded through the wide array of NLuc reporter technologies currently available. The Bioluminescence Resonance Energy Transfer (BRET) system can be implemented for in vivo use, such as for voltage and calcium sensors or deeper tissue imaging. NanoLuc can also be split into a two-part system, either for protein:protein interaction through NanoLuc® Binary Technology (NanoBiT) or the HiBiT epitope tag for a protein of interest.
Some examples of NLuc applications include the measurement of exosomes/extracellular vesicles (EVs), assessment of in vivo biodistribution of novel delivery methods (LNP) or even cell surface receptors. EVs have historically proven difficult to measure using prior BLI reporter technologies. Recent work using the NanoLuc® in vivo substrate demonstrated that there is a distinction between EV size within breast cancer models, work that was only possible through the small size of the NLuc tag (Magoling et al., 2023). Despite the many advantages of NLuc, it comes with its own challenges. NLuc emits light well below the 600 nm required for deep tissue signal penetration. These deep tissue challenges can be mitigated with several NanoLuc® fusion reporters. One example is Antares, a fusion of NanoLuc® luciferase to an orange fluorescent protein CyOFP (Su et al., 2020). The fusion of this orange fluorescent protein allows the NanoLuc® reporter to generate brighter signals that penetrate further through the tissue. BRET-based LumiFluor reporters have also been created through the fusion of NLuc with various fluorescent proteins such as green fluorescent protein or an orange fluorescent protein (Schaub et al., 2015).
Other Forms of Luciferase
There are several other luciferase reporters that are often used for in vivo studies. Renilla luciferase (RLuc) requires coelenterazine and oxygen to produce blue light at approximately 480 nm. Promega offers the ViviRen™ In Vivo Renilla Luciferase Substrate, which uses a uniquely engineered coelenterazine-based compound to minimize degradation and autoluminescence. The substrate required by RLuc allows this luciferase enzyme to pair well with others in a multiplexed assay. Recent work multiplexed RLuc with FLuc to demonstrate that xenograft tumor growth can be modulated through heat-induced miRNA expression (Pinel et al., 2018).
Another commonly used reporter would be Guassia luciferase (GLuc). It is a small (19 kDa) reporter that generates blue light (475 nm) in a burst upon exposure to both ATP and coelenterazine (Yu et al., 2018). A fast and quantitative way to measure pathways in a variety of model organisms, Guassia appears to be functional in most eukaryotic systems. Lastly, Pyrophorus plagiophtalamus (Click Beetle) is another red luciferase that requires D-luciferin. Click Beetle has demonstrated enhanced tissue penetration for both in vitro and vivo imaging.
Although this list is not exhaustive, it should shed light on the wide variety of luciferases available when designing an in vivo experiment.
Multiplexing
To maximize information gained from each animal and to further understand complex biological processes in vivo, multiplexed imaging of two reporters in the same animal can increase the rigor of an experiment. Although each reporter/substrate combination emits light at a specific wavelength, in practice deconvoluting the luminescence signal between two reporters is quite challenging. One of the most important considerations for in vivo reporter multiplexing is substrate specificity, to ensure that the bioluminescent signal read is specific to the reporter endpoint measured. FLuc and NLuc are ideal for dual imaging because there is no substrate cross-reactivity between the two enzymes. For multiplexed experiments, substrate delivery should occur sequentially to allow time for the luminescent signal to decline prior to reading the second signal. Multiplexing using several bioluminescent reporters has been demonstrated in the measurement of CAR-T cell activity (Su et al., 2020). The characteristics provided by each reporter should also be considered, such as co-factor requirements or the size of the reporter, to determine the ideal experimental set-up.
Substrate Administration
Each reporter has a distinct and specific substrate that must be introduced into the animal to generate bioluminescence. There are a variety of methods for introducing the substrate, intraperitoneal (IP) and intravenous injection (IV) injection through the tail vein being most common. IP delivery is often preferred due to ease of administration, rapid distribution in the body and increased signal stability. However, IV-administration is sometimes preferred due to a brighter initial signal, although it comes with more rapid signal decay. Figure 2 compares the normalized flux of IP and IV injection of FFz compared to IP injected D-luciferin. Additional methods such as subcutaneous and retro-orbital injections can also be used for localized substrate delivery. These substrates do not have cross-reactivity; however, as mentioned above, it is wisest to refrain from simultaneously introducing substrate for two distinct reporters due to difficulties that may arise when attempting to distinguish the two luminescent signals. Ensure that the animals are adequately prepared, which includes shaving or depilating fur in the imaging area to reduce light absorption and scattering. Finally, optimize the timing of the substrate injection (e.g., D-luciferin, FFz) to ensure peak signal intensity during imaging sessions. If multiplexing, ensure optimal time has passed before introduction of the second substrate.
Additional Considerations
Optical imaging has quickly become the standard in preclinical research. The ability to track biological activity at the molecular level enables visualization of drug biodistribution, molecular events and disease progression. A sugar-cooled charged-coupled device camera is required to detect light emission from just a few luciferase-expressing cells. Factors that directly impact the luminescent signal from within a tissue sample include the flux of the photons from the reporter, the number of functional reporter cells in the sample and the location of the reporter within the sample (Close et al., 2011). The wavelength of the reporter signal is also a consideration, as tissue absorption levels vary with the degree to which the emitted wavelength is blue shifted. If your experimental questions are best answered by deeper tissue applications (such as intraorganellar injections), consider that attenuation may occur from certain reporters. Your choice of imaging equipment is also important. Frequently a reporter read-out will include longitudinal measurements, so plan your recording setup accordingly. To properly locate bioluminescent signal inside of an animal, we recommend a multimodal approach. This refers to the pairing of optical imaging with anatomical imaging modalities, such as 2D X-ray, MRI or brightfield imaging.
Applications/Specific Example Case
A recent study illustrated the power of in vivo luciferase reporters using NanoLuc® adeno-associated viruses (AAV) to visually demonstrate viral capsid infection in living animals (Bing et al., 2023). AAV show great promise as a gene delivery mechanism. NanoLuc-expressing AAV vectors were diluted in 200 µl of PBS and injected intravenously into BALB/c mice at a dose of 1011 vg per mouse. 0.44 µmol of Nano-Glo® FFz was injected on days 8, 176, and 29. These mice were anaesthetized and imaged after 3 +/- 1 min using an IVIS spectrum imager. Imaging at 8, 17 and 29 days after infection demonstrated strong luminescence throughout the animals’ bodies compared to control animals. Through epitope modifications in an AAV9 vector, BLI demonstrated a dramatic decrease in the luminescence throughout the animal. This highlights the use of BLI in validating transduction efficiency within living models over time.
Summary
Bioluminescent imaging is an effective method for studying dynamic biological functions inside living organisms. Choosing between different reporters, each with unique advantages such as ATP independence or dependence and specific wavelength emissions, can help you tailor an experiment to your desired outcomes. Optimization is key in crafting a successful experiment and careful consideration is best applied during the planning phases. BLI is at its strongest after thoughtful selection of the reporter is paired with optimization of substrate administration and measurement. The proper application of BLI in research can lead to new and significant findings, and we encourage those interested to explore this technique further. For more information on the power of live-cell imaging, you can visit our Bioluminescence Imaging technology page.
Citations
Bing, S. J., Seirup, M., Hoang, T. T., Najera, S. S., Britten, C., Warrington, S. L., Chu, S. L., & Mazor, R. (2023). Rational immunosilencing of a promiscuous T-cell epitope in the capsid of an adeno-associated virus. Nature Biomedical Engineering. https://doi.org/10.1038/s41551-023-01129-8
Bozec, D., Sattiraju, A., Bouras, A., Jesu Raj, J. G., Rivera, D., Huang, Y., Junqueira Alves, C., Tejero, R., Tsankova, N. M., Zou, H., Hadjipanayis, C., & Friedel, R. H. (2020). Akaluc bioluminescence offers superior sensitivity to track in vivo glioma expansion. Neuro-Oncology Advances, 2 (1). https://doi.org/10.1093/noajnl/vdaa134
Close, D. M., Xu, T., Sayler, G. S., & Ripp, S. (2011). In vivo bioluminescent imaging (BLI): Noninvasive visualization and interrogation of biological processes in living animals. In Sensors (Vol. 11, Issue 1, pp. 180–206). https://doi.org/10.3390/s110100180
Kim, J. E., Kalimuthu, S., & Ahn, B. C. (2015). In Vivo Cell Tracking with Bioluminescence Imaging. In Nuclear Medicine and Molecular Imaging (Vol. 49, Issue 1, pp. 3–10). Springer Verlag. https://doi.org/10.1007/s13139-014-0309-x
Love, A. C., & Prescher, J. A. (2020). Seeing (and Using) the Light: Recent Developments in Bioluminescence Technology. In Cell Chemical Biology (Vol. 27, Issue 8, pp. 904–920). Elsevier Ltd. https://doi.org/10.1016/j.chembiol.2020.07.022
Magoling, B. J. A., Wu, A. Y. T., Chen, Y. J., Wong, W. W. T., Chuo, S. T. Y., Huang, H. C., Sung, Y. C., Hsieh, H. T., Huang, P., Lee, K. Z., Huang, K. W., Chen, R. H., Chen, Y., & Lai, C. P. K. (2023). Membrane Protein Modification Modulates Big and Small Extracellular Vesicle Biodistribution and Tumorigenic Potential in Breast Cancers In Vivo. Advanced Materials, 35 (13). https://doi.org/10.1002/adma.202208966
Mehle, A. (2015). Fiat Luc: Bioluminescence Imaging Reveals In Vivo Viral Replication Dynamics. In PLoS Pathogens (Vol. 11, Issue 9). Public Library of Science. https://doi.org/10.1371/journal.ppat.1005081
Pal, D., Blair, H., Parker, J., Hockney, S., Beckett, M., Singh, M., Tirtakusuma, R., Nelson, R., McNeill, H., Angel, S. H., Wilson, A., Nizami, S., Nakjang, S., Zhou, P., Schwab, C., Sinclair, P., Russell, L. J., Coxhead, J., Halsey, C., … Vormoor, J. (2022). hiPSC-derived bone marrow milieu identifies a clinically actionable driver of niche-mediated treatment resistance in leukemia. Cell Reports Medicine, 3 (8). https://doi.org/10.1016/j.xcrm.2022.100717
Pinel, K., Genevois, C., Debeissat, C., & Couillaud, F. (2018). Imaging of conditional gene silencing in vivo using a bioluminescence-based method with thermo-inducible microRNAs. Scientific Reports, 8 (1). https://doi.org/10.1038/s41598-018-22932-3
Refaat, A., Yap, M. L., Pietersz, G., Walsh, A. P. G., Zeller, J., del Rosal, B., Wang, X., & Peter, K. (2022). In vivo fluorescence imaging: success in preclinical imaging paves the way for clinical applications. In Journal of Nanobiotechnology (Vol. 20, Issue 1). BioMed Central Ltd. https://doi.org/10.1186/s12951-022-01648-7
Schaub, F. X., Reza, M. S., Flaveny, C. A., Li, W., Musicant, A. M., Hoxha, S., Guo, M., Cleveland, J. L., & Amelio, A. L. (2015). Fluorophore-NanoLuc BRET reporters enable sensitive In Vivo optical imaging and flow cytometry for monitoring tumorigenesis. Cancer Research, 75 (23), 5023–5033. https://doi.org/10.1158/0008-5472.CAN-14-3538
Shen, Y. T., Asthana, R., Peeters, C., Allen, C., Deangelis, C., & Piquette-Miller, M. (2020). Potential Limitations of Bioluminescent Xenograft Mouse Models: A Systematic Review. In J Pharm Pharm Sci (www.cspsCanada.org) (Vol. 23). www.cspsCanada.org
Su, Y., Walker, J. R., Park, Y., Smith, T. P., Liu, L. X., Hall, M. P., Labanieh, L., Hurst, R., Wang, D. C., Encell, L. P., Kim, N., Zhang, F., Kay, M. A., Casey, K. M., Majzner, R. G., Cochran, J. R., Mackall, C. L., Kirkland, T. A., & Lin, M. Z. (2020). Novel NanoLuc substrates enable bright two-population bioluminescence imaging in animals. Nature Methods, 17 (8), 852–860. https://doi.org/10.1038/s41592-020-0889-6
Yu, T., Laird, J. R., Prescher, J. A., & Thorpe, C. (2018). Gaussia princeps luciferase: a bioluminescent substrate for oxidative protein folding. Protein Science, 27 (8), 1509–1517. https://doi.org/10.1002/pro.3433
Learn More
The Nano-Glo® Fluorofurimazine In Vivo Substrate (FFz) is optimized for in vivo detection of NanoLuc® luciferase, NanoLuc® fusion proteins or reconstituted NanoBiT® luciferase.
Related Resources
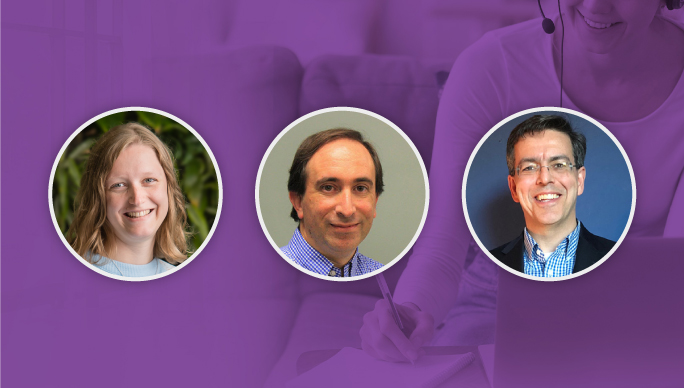
Mastering Bioluminescent Imaging for Dynamic Biological Studies
Hear from our featured experts, Dr. Thomas Kirkland (Promega) and Dr. Andrew Van Praagh (Spectral Instruments Imaging), in this webinar.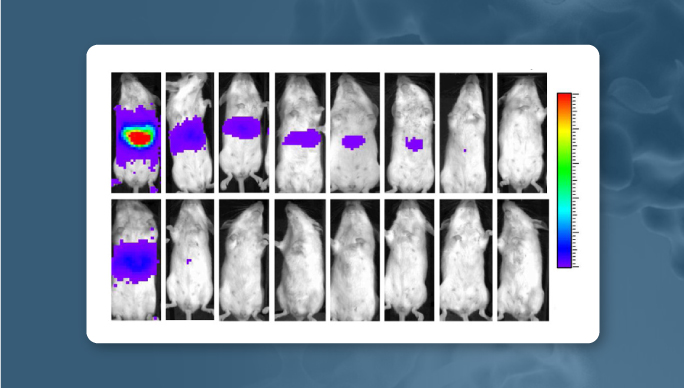
Live-Cell Bioluminescent Imaging
Learn more about in vivo imaging with NanoLuc® Luciferase.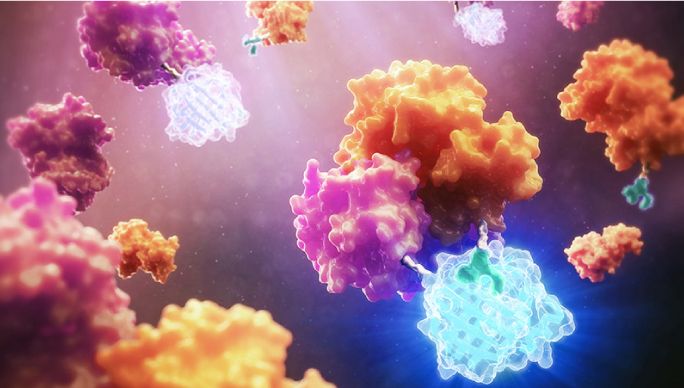