Exploring Gene Expression Through siRNA and miRNA Transfection
Introduction
Regulation of gene expression is extremely important for development and maintenance of cellular function. RNA-mediated genetic regulation utilizes several types of small RNAs, such as small interfering RNA (siRNA) and micro-RNA (miRNA) for targeted genetic knockdown (1). Organisms ranging from fungi to humans rely on this type of genetic regulation. Thus, understanding how this form of regulation works provides insights into a highly conserved mechanism across evolutionary history (2).
The mode of action of these small RNAs are quite similar. Both single stranded miRNA and double-stranded siRNA utilize an RNA-induced silencing complex (RISC) to regulate target mRNA sequences (3). miRNA competitively inhibits through complementary sequence binding in the 3’ upstream element of their target messenger-RNA (mRNA) (4). This binding to mRNA typically leads to target degradation or suppression upon shortening of the mRNA poly(A) tail and the removal of the 5’ cap end structure. Distinct from miRNA, siRNA strands are separated and the more stable 5’-end strand is integrated into RISC (3). This remaining siRNA strand aligns the RISC complex with the target messenger RNA (mRNA) where Ago-2, a protein found in RISC, mediates cleavage of the mRNA and thus target inhibition (1). siRNA can facilitate very efficient gene knockdown through very low concentrations (picomolar range) as the siRNA-loaded RISC utilizes a catalytic mechanism of binding, dissociation, followed by binding to facilitate the degradation of multiple mRNA with one RISC complex (1). siRNA also inhibits only the expression of one specific target mRNA. In contrast, miRNA tends to target several different mRNA sequences due to the relatively short ‘seed sequence’ found on the miRNA, thus providing a more expansive knockdown effect.
The introduction of either siRNA or miRNA directly into cells results in knockdown of target genes. This provides an opportunity to fine-tune genetic expression and study the requirement of specific mRNA. This has broader implications for identifying the function of various genes in larger cellular processes such as cellular signaling, cancer progression, and cellular apoptosis.
Small RNA Transfection
The first step of successfully utilizing either siRNA or miRNA is to get these small sequences into the cell. Cell transfection is routinely used in vitro and facilitates the introduction of genetic materials into cells to modulate the activity or expression of proteins of interest (5). Transfection of small molecules such as siRNA or miRNA into cells can induce genetic knockdown of target mRNA sequences to lower protein expression. By themselves, both miRNA and siRNA structures are unlikely to become incorporated into a cell due to various features, such as short half-lives, and charges that natively repel them from the negatively charged cell membrane (6). Transfection reagents provide safe transport across the lipid bilayer of the cell membrane. A lipid-based delivery system is typically incorporated into transfection reagents as liposomes form a coated vesicle to protect the small RNA as it crosses the membrane (3). Liposomes are the most widely studied gene carriers due to their biodegradability and biocompatibility (6). The FuGENE® SI Transfection Reagent provides maximum efficiency and gentle small RNA transfection to achieve superior gene silencing with minimal to no toxicity.
The use of small RNA for genetic knockdown has many applications within cell research. Both miRNA and siRNA can be used for general gene silencing, functional genomics, cellular analysis, constructing RNA library screens and more. Several exciting categories of research using these small RNA tools are outlined below.
siRNA Screening
One of the most useful applications of siRNA is its use in unbiased functional genomic screens. These large screens provide an opportunity to identify fundamental biological pathways and regulators involved in often complex cellular conditions. A recent study conducted by Pai et al. explored Fanconi anemia, a rare chromosomal-instability syndrome where individuals have increased risk of head-and-neck squamous-cell-carcinomas. Within the context of this complicated disease, this study identified novel genes that could act as therapeutic targets for targeted intervention, such as RBBP9, and presented these genes through a publicly available dataset (7). Work such as this is important as it can identify potentially druggable candidate genes that may be missed through more targeted identification procedures.
Cell Signaling
siRNA research has been employed to study a variety of cellular processes. Cells utilize various signaling processes to convey messages regarding stress, metabolism or growth. Recent work utilizing siRNA repressed specific subunits of the mammalian cleavage factor I (CFIm) and demonstrated that over 800 genes undergo alternative polyadenylation (APA) (8). APA is the main contributor to transcriptome diversity; thus, this work indicates that siRNA can facilitate the generation of large datasets of downstream regulation through changes in signaling pathways. Another example would be CD-38, a gene involved in acquired resistance of T-cells. Wang et al. showed that jeopardized immunotherapy may be supplemented through reactive oxygen species mediated siRNA gene knockdown (9). Complex conditions such as intracranial aneurysm involve the signaling pathway AMPK/NF-kB. Recent work has shown that miR-323a-3p regulates both this pathway and the accumulation of inflammatory factors (IL-1β) in this disease state (10). Tight regulation of cell signaling is vital to maintain cellular homeostasis. As our understanding of genes involved in these pathways continues to improve, siRNA and miRNA provide ample opportunity to modify these targets to improve our understanding of the cell.
Cancer
Cancer is the second leading cause of death in the world (11). As our understanding of genetic pathways improves, we can explore regulation of those pathways through administration of siRNA and miRNA. Recent work has utilized siRNA to identify components of the PI3K/AKT/mTOR signaling axis within cancer models. A study from the University of Queensland, Brisbane, identified ORAI1 as a possible therapeutic target in cancer cells through their siRNA-mediated silencing of PTEN (12). The study highlighted how certain activity in cancer cells, such as abnormal AKT signaling and calcium signaling, can be attenuated by targeted suppression of predicted genetic targets upstream. Separate evidence further demonstrated the power of siRNA modulation of the PI3K/AKT/mTOR pathway, where knockdown of REDD1 was shown to improve the anticancer activity of aspirin in a subset of breast cancers (13). Substantial work has also explored the role of miRNA within cancer progression. Once identified, genetic pathways involved in cancer progression can be similarly modulated through changes in predicted miRNA regulators. A great example of this would be miR-326, which appears to have a tumor-suppressive role through its inhibition of the ErbB/PI3K pathway (14). This pathway appears to be involved in the progression of breast cancer development, therefore miRNA may provide a possible avenue for treating this type of cancer.
Apoptosis
One of the ways we can improve our understanding of apoptosis is through targeted suppression of identified/predicted apoptotic genes by both siRNA and miRNA. Wang et al. aimed to determine the role of Ndufs4, a component of the mitochondrial complex I, in regulating apoptosis. First they used siRNA to selectively knockdown Ndufs4, reducing mRNA and protein levels by 76.7% and 64.9% respectively (15). The apoptosis rate of these treated cells was significantly lower than control-treated cells, indicating that upregulation of this gene is vital for apoptosis to occur.
Lung cancer has the highest morbidity and mortality of all cancer types (16). Studies using siRNA have suggested a role of GINS complex subunit 2 (GINS2) in the cell proliferation and apoptosis associated with lung cancer (16). Knockdown of GINS2 using siRNA showed a significant increase in the apoptosis rate as well as increases in p53 levels. To further understand apoptosis, researchers can study the miRNAs predicted to regulate important pathways. Recent work has identified miR-29b as important within apoptosis, where it was shown to inhibit prostate growth in mice through induction of apoptotic gene BCL2L11 (17). These studies solidify the importance of using small RNAs to understand mechanisms involved in apoptosis and possible therapeutics.
Clinical Applications
Despite the challenges of utilizing siRNA within the clinical realm, there has been significant progress in siRNA drug development within recent years. Between 2018 and 2022, five siRNA drugs were approved for use in humans, marking a major milestone in the use of siRNA in therapeutics. All five of these drugs, Patisiran, Givosiran, Lumasiran, Inclisiran, and Vutrisiran, target mRNA expressed in the liver (18). The liver is easy to target as siRNAs introduced into the patient tend to accumulate there for detoxification. Due to this rapid accumulation in the liver, different avenues for delivery are required for mRNA targets not found in the liver. Treatment of osteoporosis, a disease characterized by decreased bone mass and destruction of bone microarchitecture, is currently being explored through bacterial derived extracellular vesicles (BEVs), as they facilitate targeted delivery of siRNA agents (19).
Conclusion
As our understanding of genetic regulation deepens, research exploring endogenous mechanisms will continue to expand. Due to the inherent fine-tuned regulation through which both siRNA and miRNA engage with their target mRNA, a large swath of work remains to be done within this field. In the lab, genetic knock-down using small RNA provides a precise avenue for understanding regulatory pathways, potentially contributing to a better understanding of the world at large.
References
- Friedrich, M. & Aigner, A. (2022) Therapeutic siRNA: State-of-the-art and future perspectives. BioDrugs. 36(5), 549–571.
- Ha, M. et al. (2008) Interspecies regulation of microRNAs and their targets. Biochim. Biophys. Acta - Gene Regul. Mech. 1779(11), 735–742.
- Dana, H. et al. (2017) Molecular mechanisms and biological functions of siRNA. Int J Biomed Sci. 13(2).
- Cai, Y. et al. (2009) A brief review on the mechanisms of miRNA regulation. Genom. Proteom. Bioinform. 7(4), 147–154.
- Cocchiararo, I. et al. (2022) Back to basics: Optimization of DNA and RNA transfer in muscle cells using recent transfection reagents. Exp. Cell Res. 421(2).
- Xia, Y. et al. (2016) Effect of surface properties on liposomal siRNA delivery. Biomaterials. 79, 56–68.
- Pai, G. et al. (2023) Genome-wide siRNA screens identify RBBP9 function as a potential target in Fanconi anaemia-deficient head-and-neck squamous cell carcinoma. Commun. Biol. 6(1).
- Ghosh, S. et al. (2022) CFIm-mediated alternative polyadenylation remodels cellular signaling and miRNA biogenesis. Nucleic Acids Res. 50(6), 3096–3114.
- Wang, X. et al. (2022) T cell-signaling-responsive conjugate of antibody with siRNA to overcome acquired resistance to anti-PD-1 immunotherapy. Adv. Ther. 5(1).
- Sun, B. et al. (2022) miRNA-323a-3p promoted intracranial, aneurysm-induced inflammation via AMPK/NF-κB signaling pathway by AdipoR1. Adv. Clin. Exp. Med. 31(11).
- Ali, L.M.A. & Gary-Bobo, M. (2022) Photochemical internalization of siRNA for cancer therapy. Cancers 14(15).
- Bong, A.H.L. et al. (2022) AKT regulation of ORAI1-mediated calcium influx in breast cancer cells. Cancers 14(19).
- Savukaitytė, A. et al. (2021) siRNA knockdown of redd1 facilitates aspirin-mediated dephosphorylation of mtorc1 target 4e-bp1 in mda-mb-468 human breast cancer cell line. Cancer Manag. Res. 13, 1123–1133.
- Ghaemi, Z. et al. (2019) MicroRNA-326 Functions as a tumor suppressor in breast cancer by targeting ErbB/PI3K signaling pathway. Front. Oncol. 9.
- Wang, B. et al. (2023) Recombinant adenovirus siRNA knocking down the Ndufs4 gene alleviates myocardial apoptosis induced by oxidative stress injury. Cardiol. Res. Pract.
- Chi, F. et al. (2020) Knockdown of GINS2 inhibits proliferation and promotes apoptosis through the p53/GADD45A pathway in non-small-cell lung cancer. Biosci. Rep. 40(4).
- Sur, S. et al. (2019) MiRNA-29b inhibits prostate tumor growth and induces apoptosis by increasing bim expression. Cells 8(11).
- Ahn, I. et al. (2023) Where should siRNAs go: Applicable organs for siRNA drugs. Exp. Mol. Med. 55(7), 1283–1292.
- Liu, H. et al. (2023) Bone-targeted bioengineered bacterial extracellular vesicles delivering siRNA to ameliorate osteoporosis. Compos. Part B: Eng. 255.
Learn more about the FuGENE® SI Transfection Reagent.
Related Resources
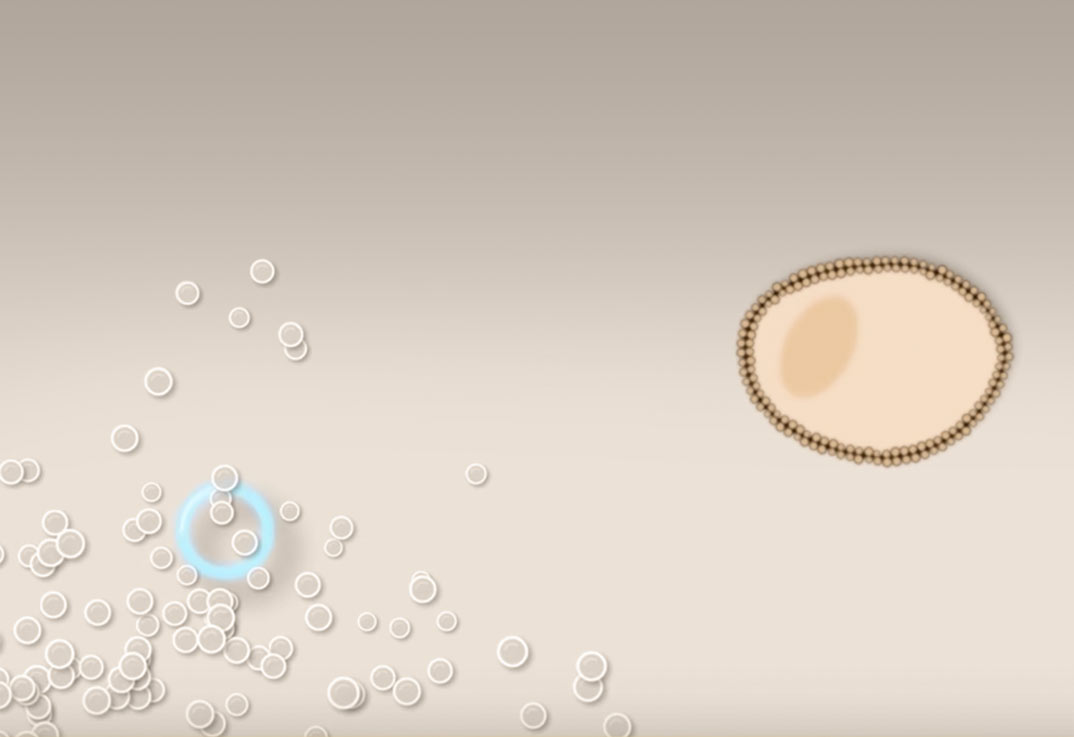
Transfection Guide
This guide provides an overview of transfection methods, example protocols and guidance for choosing a transfection reagent.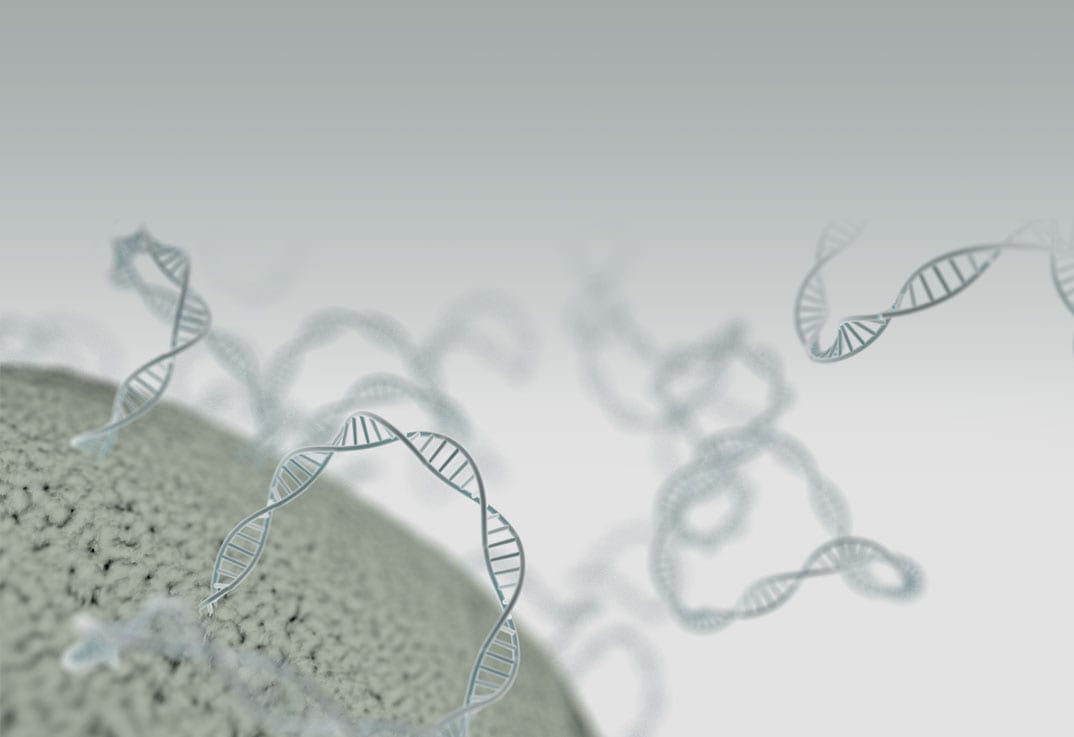
Article: Optimize Transfection of Cultured Cells
Learn how to optimize transfection conditions and what factors influence success.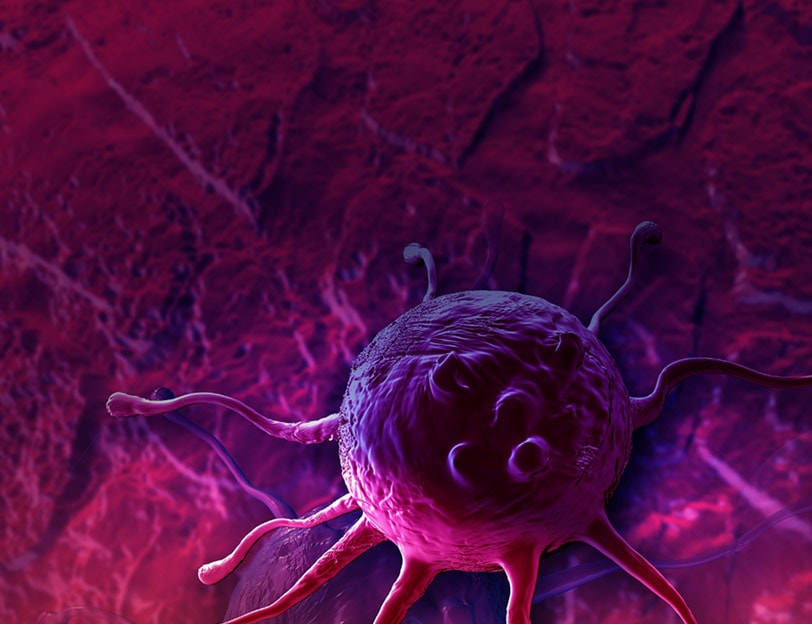