Comparison of luciferases as ATP Sensors in the presence of inhibitors: CellTiter-Glo® Assay shows less compound interference than the Perkin Elmer ATPlite™ 1step assay
Promega Corporation
Publication Date: 2011
Abstract
Reducing the number of false positives in a high-throughput screen is a key goal for any researcher trying to minimize downstream efforts. Luciferase-based systems are used widely as reporters for drug screens; however, compounds in a library can inhibit luciferase, resulting in false positives. Ultra-Glo™ rLuciferase, an evolved luciferase from the firefly, Photuris pennsylvanica, is less sensitive to compound inhibition than wild-type firefly luciferase. In this study, we directly compare the abilities of the Promega CellTiter-Glo® Luminescent Cell Viability Assay, an Ultra-Glo™ rLuciferase-based luminescent ATP detection system, and the Perkin Elmer ATPlite™ 1step assay to resist common commercial luciferase inhibitors. The CellTiter-Glo® Assay has greater than 80% activity for 5 out of the 7 compounds at an inhibitor concentration of 10µM; whereas, ATPlite™ 1step has 80% activity for only 1 out of 7 compounds at the same inhibitor concentrations.
Introduction
High-throughput screening (HTS) is widely used by the pharmaceutical industry for drug discovery. It leverages automation to quickly assay the biological or biochemical activity of a large number of drug-like compounds and is useful for discovering ligands for receptors, enzymes, ion-channels or other pharmacological targets, or for profiling cellular or biochemical pathways. Firefly luciferase is a common reporter used in HTS because of its sensitivity, ease of use, and low expense(1). Firefly luciferase can be used to measure ATP concentrations, as a reporter of cell-based gene expression and to assay the turnover of proluciferin substrates in the presence of target enzymes. High-throughput, luciferase-coupled assays do not require expensive antibodies or fluorescent labels and therefore provide a platform for screening that reduces assay development time and reagent costs.
One important consideration in any assay that uses an enzymatic reporter is inhibition of that reporter enzyme by compounds from the library(1). Auld et al. found that approximately 3% of a 72,000-compound library repressed Photinus pyralis luciferase, of which 681 (0.9%) exhibited IC50 < 10µM(2) (3). Ultra-Glo™ rLuciferase, an optimized luciferase derived from the firefly Photuris pennsylvanica, was created by directed evolution and formulated to generate a stable “glow-type” luminescent signal and improve performance across a wide range of assay conditions. Using Ultra-Glo™ rLuciferase, only 0.1% of compounds tested showed an IC50 < 10µM compared to 0.9% found from the same study with firefly luciferase from Photinus pyralis (lucPpy); therefore, Ultra-Glo™ rLuciferase shows less compound interference when used as an ATP sensor compared to lucPpy(3).
In this report, we compare the resistance of two different luminescence ATP detection systems, CellTiter-Glo® Cell Viability Assay and ATPlite™ 1step assay, to known luciferase inhibitors. Both CellTiter-Glo® and ATPlite™ 1step assays are ATP-monitoring systems based on firefly luciferase (evolved Ultra-Glo™ rLuciferase from Photuris pennsylvanica for the CellTiter-Glo® Assay and Photinus pyralis for the ATPlite™ 1step assay). Both assays are homogeneous, high-sensitivity ATP-monitoring, single-addition assays for the quantification of viable cells. All compounds tested are available from commercial sources (Table 1). Resveratrol, EMD1 (Luciferase Inhibitor 1, EMD), MPEP, SIB 1893 and pifithrin-α, are all noncompetitive inhibitors with respect to luciferin and ATP(4) (5); whereas, EMD2 (luciferase Inhibitor 2, EMD) and luciferin-6 methyl ether are competitive inhibitors with respect to luciferin. We determined the IC50 values for each assay and inhibitor.
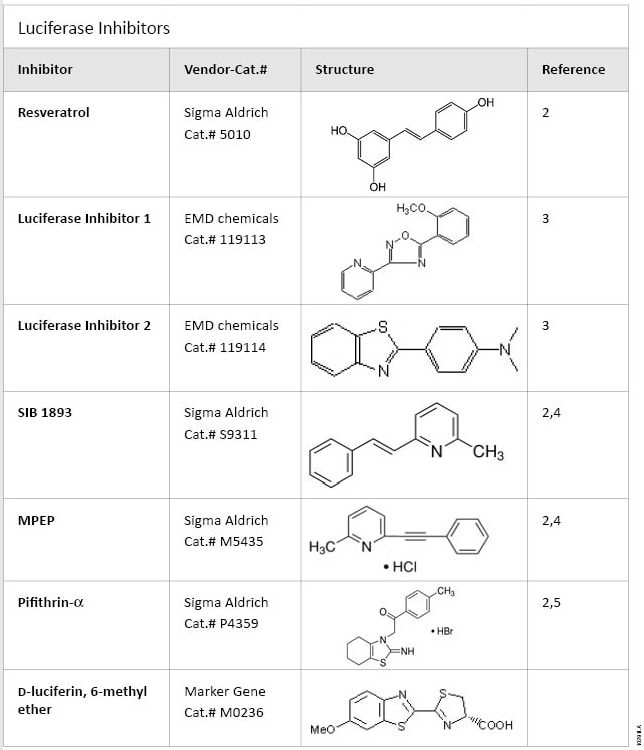
Source and structure of luciferase inhibitors used to compare sensitivity of the CellTiter-Glo® and the ATPlite™ 1step Assays to compound inhibition.
Materials and Methods
Inhibitors: Seven known luciferase inhibitors were purchased from various vendors as indicated in Table 1. DMSO was used as a solvent for each compound and was used in subsequent serial dilutions.
Luminescent ATP Detection Assay Systems: Two commercial luminescent ATP detection systems were used: CellTiter-Glo® Luminescent Cell Viability Assay (Cat.# G7570), and ATPlite™ 1step (Perkin Elmer Cat.# 6016736). Reagents were prepared as described in the respective technical manuals.
Assay for inhibition of ATP-based luminescence: In a white 96-well plate (Costar® 96-well solid white plates, tissue culture treated, sterile Cat.# 3917), luciferase inhibitor and buffer (40mM Tris [pH 7.5], 2mM MgCl2, 0.1mg/ml BSA) were added to a final volume of 90µl. The compounds were diluted serially in DMSO and added at a final concentration of 400µM to 0.025µM in fivefold increments. The final DMSO concentration in each well after addition of luminescent ATP detection reagent was 1%. A total of 100µl of the luminescent ATP detection reagent was added to each well. After a brief mix on a plate shaker, the reactions were incubated at room temperature for 10 minutes. A volume of 10µl of 10µM ATP was added to each well, and the plate was mixed for 30 seconds on a plate shaker. Luminescence was measured after two minutes and 10 minutes using a GloMax®-Multi+ Detection System with Instinct™ Software (Cat.# E9032). A DMSO vehicle control was used for background subtraction, and reactions were performed in replicates of six. Data were graphed using GraphPad Prism® software.
Assay for inhibition of cell-based luminescence: In a white 96-well plate (Costar® 96-well solid white plates, tissue culture treated, sterile Cat.# 3917), compounds and 100µl of luminescent ATP detection reagent were added to a final volume of 110µl. The compounds were diluted serially in DMSO and added at a final concentration of 400µM to 0.025µM in fivefold increments. The final DMSO concentration in each well after addition of luminescent ATP detection reagent was 1%. After a brief mix on a plate shaker, the reactions were incubated at room temperature for 10 minutes. A volume of 90µl of Jurkat cells (2.2 × 105 cells/ml; 20,000 cells/well) was added to each well, and the plate was mixed for 30 seconds on a plate shaker. Luminescence was measured at two minutes and 10 minutes using a GloMax®-Multi+ Detection System with Instinct™ Software (Cat.# E9032). A DMSO vehicle control was used for background subtraction, and reactions were performed in replicates of six. Data were graphed using GraphPad Prism® software.
Results
To determine the effect of compounds on the CellTiter-Glo® Assay (CTG) and the Perkin Elmer ATPlite™ 1step assay (PE), we first wanted to test the assays under minimal conditions by including only buffer, ATP and luminescent reagent. In order to select an appropriate ATP concentration for both assays, we created an ATP titration curve with both reagents according to the manufacturers’ protocols (data not shown). We chose 0.5µM ATP final concentration because it gave high signal and fit within the linear range of both assays. Buffer, luciferase inhibitor and luminescent reagent were mixed, incubated and then ATP was added. The test compounds were titrated in order to create a curve and determine IC50 values. Luminescence was detected, and the data were processed. The two-minute data points were used for the PE samples, and the 10-minute data points were used for the CellTiter-Glo® Assay samples, as dictated by the manufacturers’ protocols. Background for each assay was determined by adding water instead of ATP. The vehicle control (DMSO with no inhibitor) was used as the uninhibited control and set at 100%. All inhibitor data sets are displayed as a percentage of the vehicle control (Figure 1A). All the curves for the CellTiter-Glo® Assay are shifted to the right compared to the ATPlite™ 1step assay, indicating higher IC50 values; therefore, they were more resistant to the inhibitors. IC50 values were calculated using GraphPad Prism® software and plotted (Figure 1B). All compounds had a greater effect on the ATPlite™ 1step assay than the CellTiter-Glo® Assay. One compound concentration commonly used in compound screens is 10µM. Since we did not directly test at 10µM, we extrapolated the percent luciferase activity at that concentration using graphs generated by GraphPad Prism® software (Figure 1C). The CellTiter-Glo® Assay had greater than 80% activity for 5 out of the 7 compounds at 10µM; whereas Perkin Elmer's ATPlite™ 1step assay had 80% activity for only 1 out of 7 compounds.

Panel A. Graph showing inhibition of luciferases in the CellTiter-Glo® (CTG) and ATPlite™ 1step (PE) luminescent assays. Data are represented as a percent of the DMSO vehicle control set as 100%. Concentrations are displayed as M (molar). Red curves correspond to CellTiter-Glo® Assay, and blue curves correspond to ATPlite™ 1step Assay (PE). Error bars represent the standard deviation of six replicates. Panel B. IC50 values (µM) calculated using GraphPad Prism® software. Any value above 50µM is represented as >50. Panel C. Graph showing extrapolated percent activity of luciferase in either CellTiter-Glo® or ATPlite™ Assay at an inhibitor concentration of 10µM. Red bars correspond to CellTiter-Glo® Assay, and blue bars correspond to ATPlite™ 1step Assay (PE).
To determine if the amount of ATP affects the calculated IC50 values, the experiments were repeated with 0.5nM ATP (1000-fold less), a concentration still within the linear range of both luminescent assays (data not shown). The graphs and calculated IC50 values did not change using less ATP, indicating that ATP levels do not effect the inhibition and calculated IC50 values. This also suggests that these inhibitors are noncompetitive with respect to ATP.
For a more practical comparison of the ability for each luminescent reagent to resist known inhibitors, we used live cells. Live cells create ATP, and upon lysis by the reagent, ATP is released and is used to drive the luciferase reaction. Luminescent reagent and compound were mixed, then 20,000 Jurkat cells were added to each reaction. The resulting luminescence was detected and graphed as described earlier (Figure 2A). Once again, the CellTiter-Glo® assay curves are shifted to the right compared to the ATPlite™ 1step assay, indicating greater IC50 values. The IC50 values were calculated and reveal that the CellTiter-Glo® assay is more resistant to these compounds than ATPlite™ 1step assay (Figure 2B). At 10µM inhibitor, the CellTiter-Glo® assay has greater than 80% activity for five out of the seven compounds; whereas, the ATPlite™ 1step assay has 80% activity for only 1 out of 7 compounds (Figure 2C). We tested another luminescent reagent that also contains Ultra-Glo™ rluciferase and found that it has similar IC50 values as CellTiter-Glo® assay, suggesting that this increase in resistance to compound interference is probably from Ultra-Glo™ rluciferase and not from other components in the buffers and reagents (data not shown).

Panel A. Graph showing inhibition of luciferases in the CellTiter-Glo® (CTG) and ATPlite™ 1step (PE) luminescent assays. Data are represented as a percent of the DMSO vehicle control, set as 100%. Concentrations are displayed as M (molar). Red curves correspond to CellTiter-Glo®, and blue curves correspond to PE. Error bars represent the standard deviation of six replicates. Panel B. IC50 values (µM) calculated using GraphPad Prism® software. Any value above 50µM is represented as >50. Panel C. Graph showing percent activity of luciferase in either CellTiter-Glo® or Perkin Elmer (PE) at an inhibitor concentration of 10µM. Red bars correspond to CellTiter-Glo® Assay, and blue bars correspond to ATPlite™ 1step assay (PE).
Conclusions
The Ultra-Glo™ rluciferase in the CellTiter-Glo® Assay exhibited less compound interference when used as an ATP sensor compared to the lucPpy in the Perkin Elmer ATPlite™ 1step assay. When performing drug screening activities, Ultra-Glo™-based luciferase systems will result in lower false positives because of this increased resistance to compound interference.
Related Products
Related Protocols
Related Resources
Article References
- Thorne, N., Auld, D.S. and Inglese, J. (2010) Apparent activity in high-throughput screening: origins of compound-dependent assay interference. Curr. Opin. Chem. Biol. 14, 315–24.
- Auld, D.S. et al. (2008) Characterization of chemical libraries for luciferase inhibitory activity. J. Med. Chem. 51, 2372–86.
- Auld, D.S. et al. (2009) A basis for reduced chemical library inhibition of firefly luciferase obtained from directed evolution. J. Med. Chem. 52, 1450–8.
- Hawkins, E. et al. (2007) ONE-Glo™ Luciferase Assay System: New substrate, better reagent. Promega Notes 97, 30–2.
- Rocha, S. et al. (2003) The p53-inhibitor pifithrin-alpha inhibits firefly luciferase activity in vivo and in vitro. BMC Mol. Bio. 4, 9.
How to Cite This Article
Scientific Style and Format, 7th edition, 2006
Hook, B. and Schagat, T. Comparison of luciferases as ATP sensors in the presence of inhibitors: CellTiter-Glo® Assay shows less compound interference than the Perkin Elmer ATPlite™ 1step assay. [Internet] 2011. [cited: year, month, date]. Available from: https://www.promega.com/es-es/resources/pubhub/celltiter-glo-assay-shows-less-compound-interference-than-other-luminescent-assays/
American Medical Association, Manual of Style, 10th edition, 2007
Hook, B. and Schagat, T. Comparison of luciferases as ATP sensors in the presence of inhibitors: CellTiter-Glo® Assay shows less compound interference than the Perkin Elmer ATPlite™ 1step assay. Promega Corporation Web site. https://www.promega.com/es-es/resources/pubhub/celltiter-glo-assay-shows-less-compound-interference-than-other-luminescent-assays/ Updated 2011. Accessed Month Day, Year.